Introduction
This study investigates the biomechanics of the freestyle kayaking clean front loop about the transverse axis. It considers the kinematic and kinetic parameters which define the take-off, flight and landing phases (shown in Figure 2). In order to determine the optimum body configuration for the take-off and flight phases the same athlete (GB Freestyle Team member) performed three variations of the front loop;
Loop 1 – Full flexion of the arms throughout take-off and flight.
Loop 2 – Full flexion of the arms during take-off, and shoulder abducted to 90°, externally rotated to 90º (in the frontal plane) and the elbow flexed to 90º for flight and landing.
Loop 3 – Arms remain stationary, shoulder abducted to 90°, externally rotated to 90º (in the frontal plane) and the elbow flexed to 90º, during the take-off and flight phases.
The loops are shown below in Figure 1.



Figure 1 – Stick-figure showing looping sequence for technical variation in loop 1, 2 and 3.
The study considered the impact of these variations upon height, rotation and recovery in order to provide interventions to improve performance. This is important to the performance of the clean front loop as additional points can be obtained for height and not using the paddle during the initiation phase.
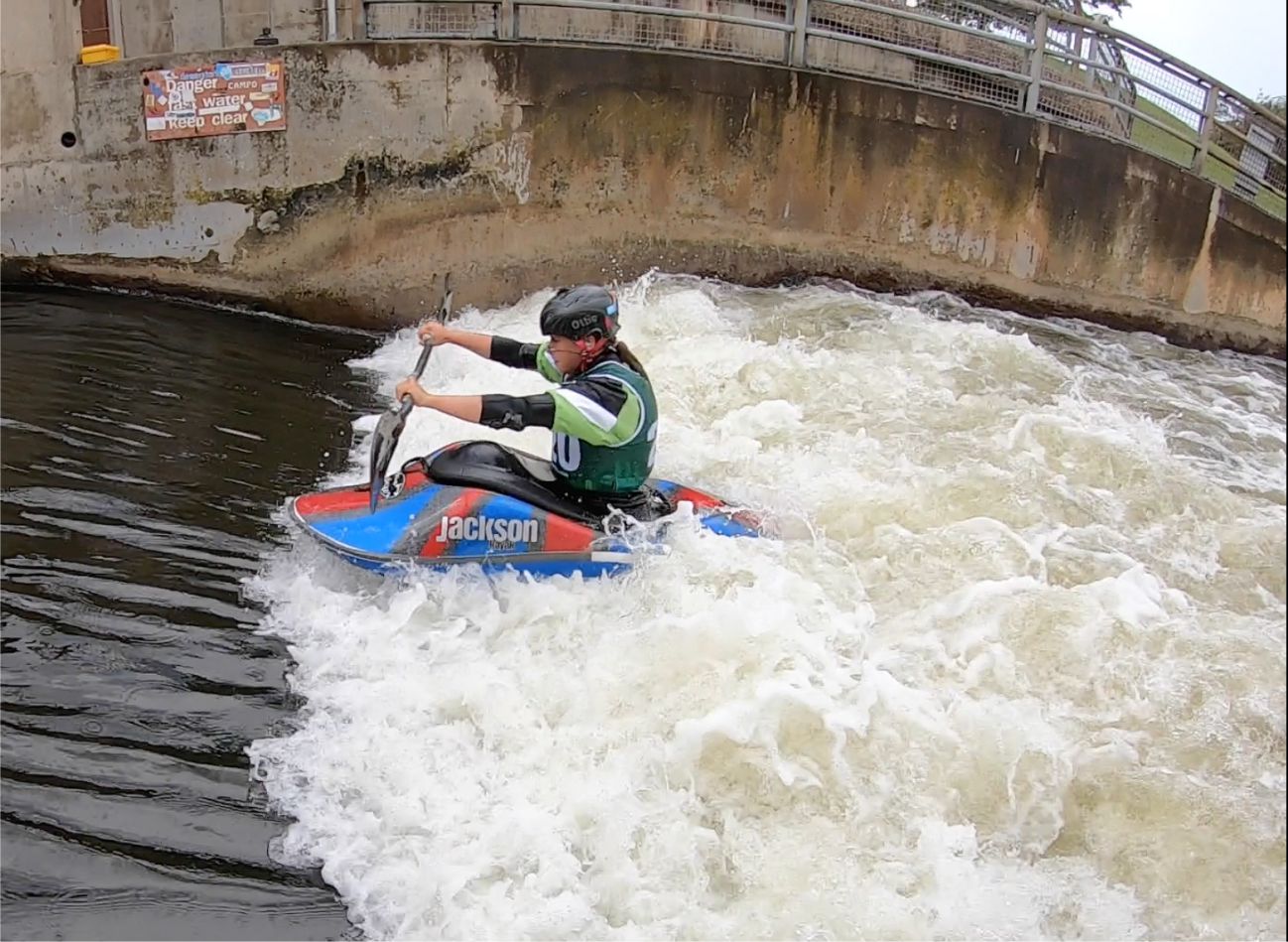



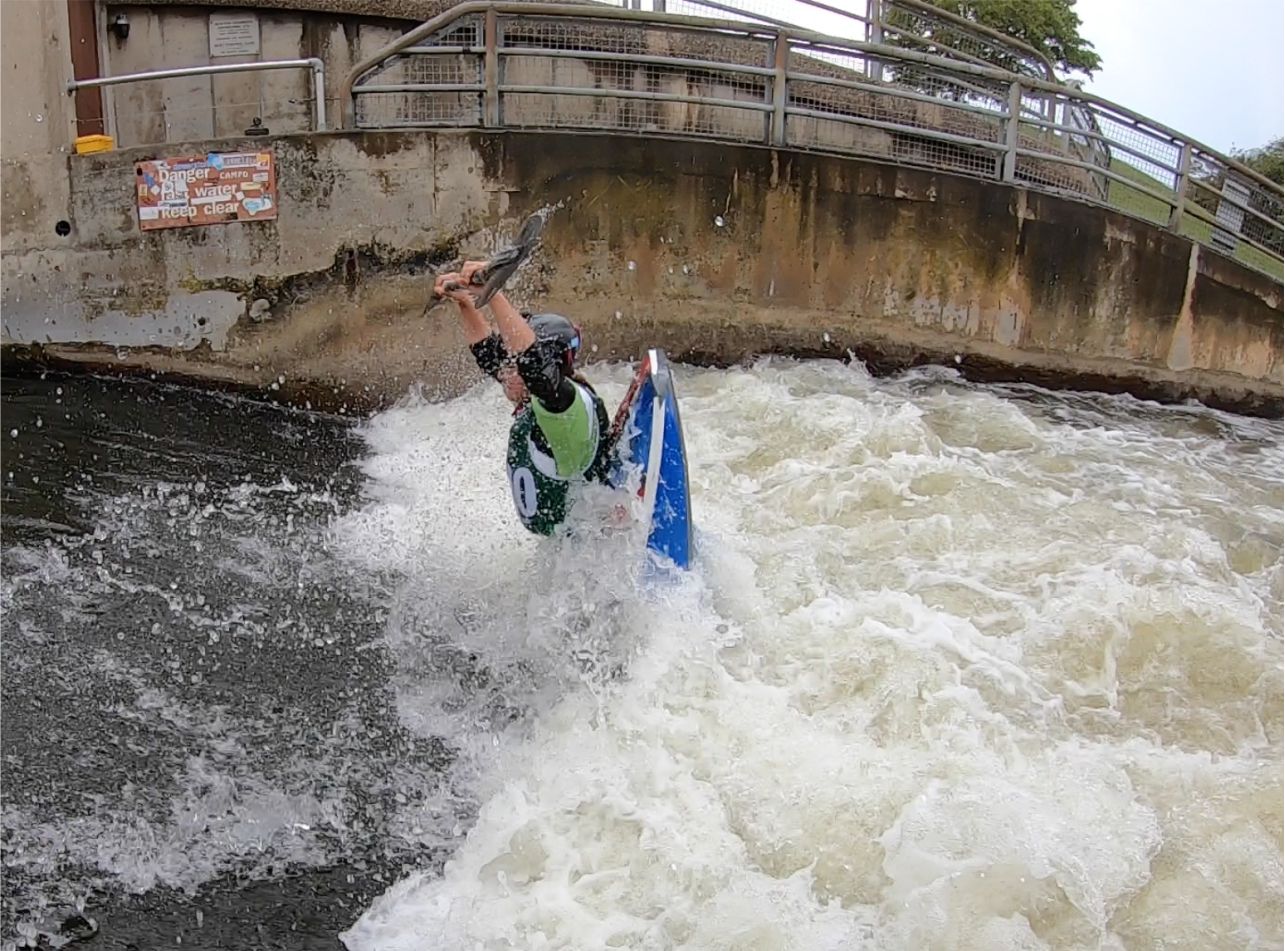
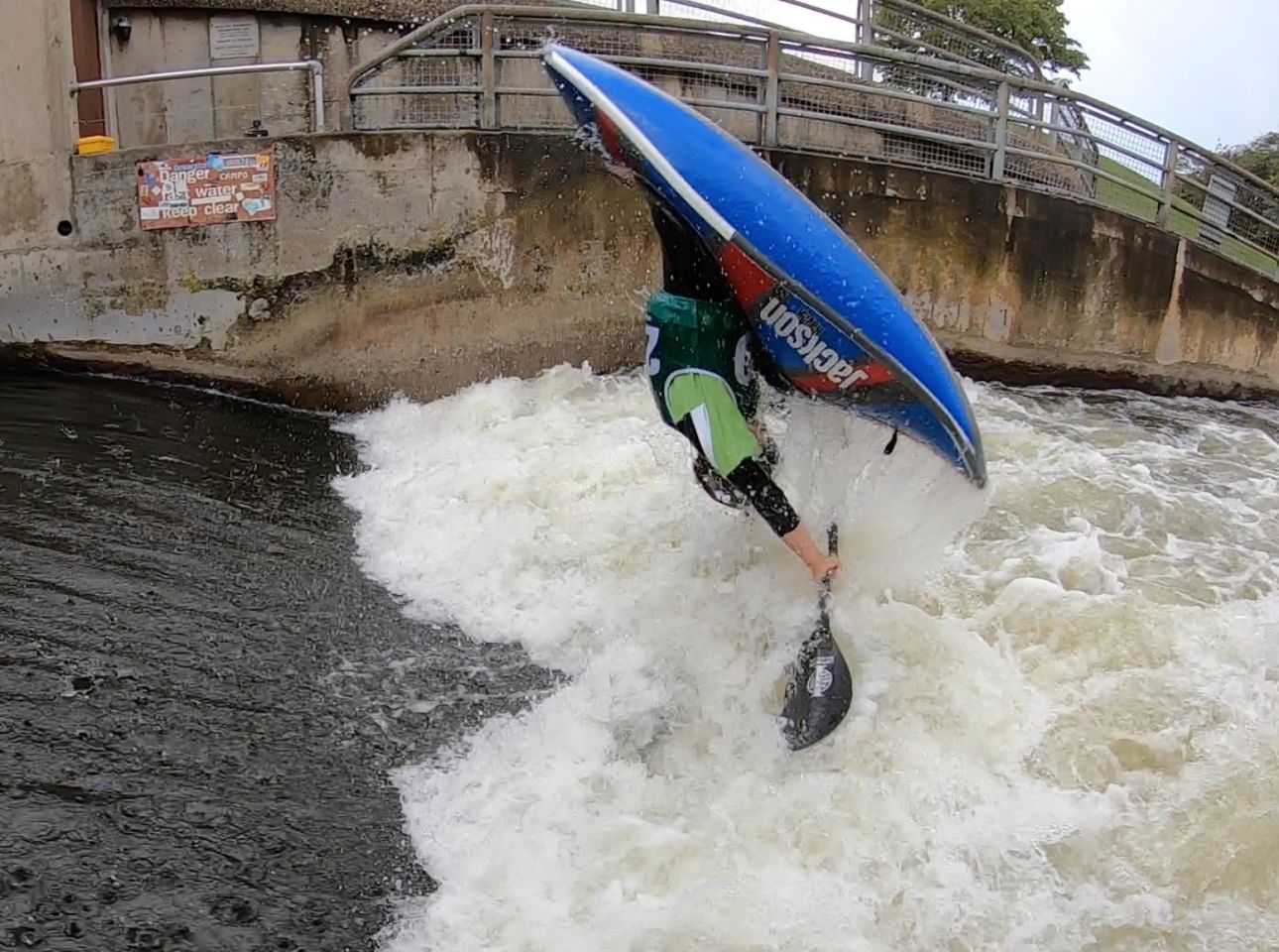
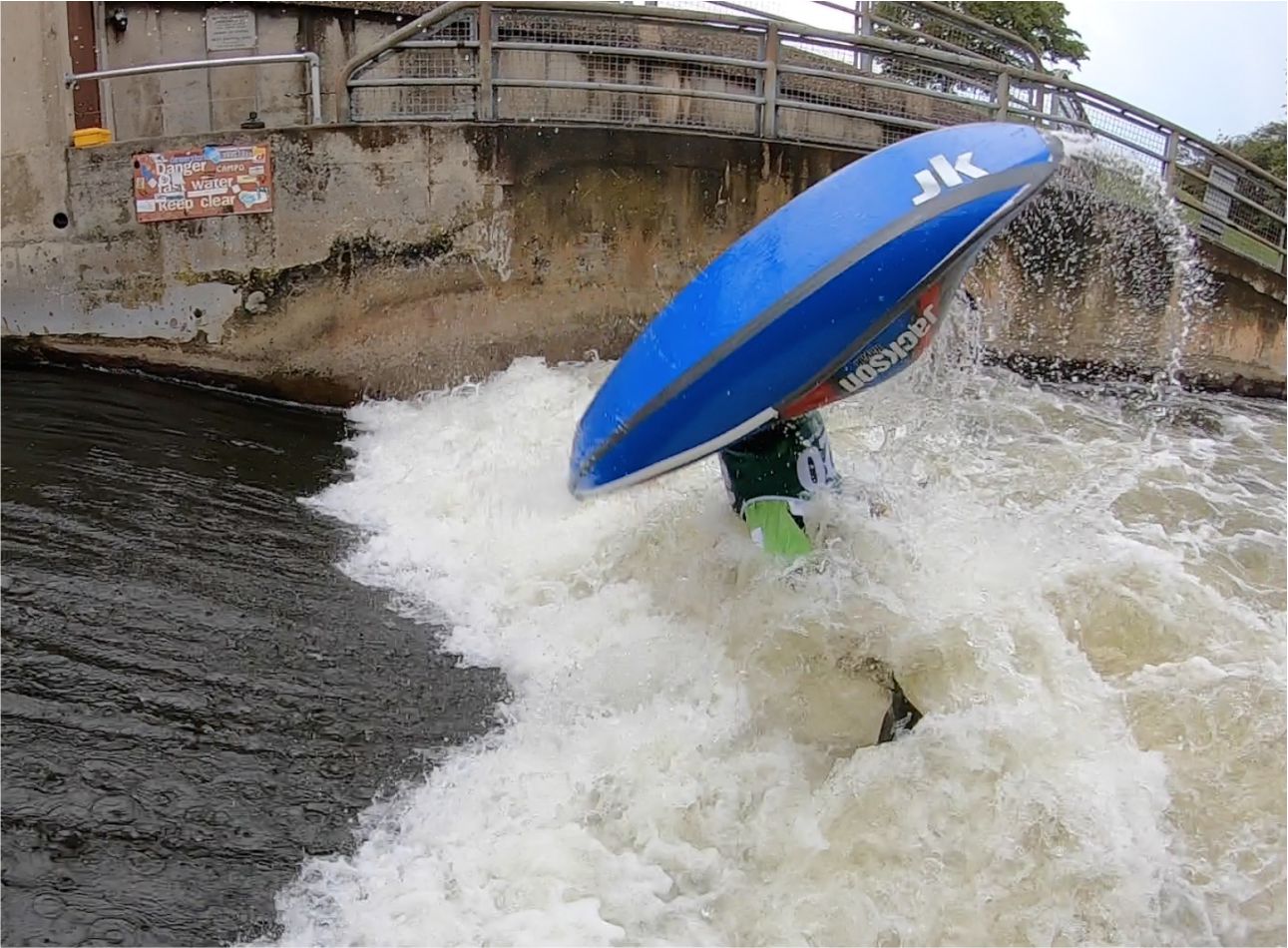

Figure 2, Key events of a Front Loop; 1) Set-up, the boat travels upstream with linear momentum, 2) Initiation, as the boat submerges the athlete begins to lean backwards, 3) Takeoff, after a quick stand-and-reach, the paddler tucks aggressively forwards to start the looping motion, 4) Recovery, paddler extends the body briefly to push down with their feet and complete the loop.
There is no current research into the kinematics of the freestyle kayak and comparisons have been taken from spring board diving (Sanders and Wilson, 1987).
Method
The participant was a 30 year old female kayaker, selected from the GB freestyle kayak team. The tests were conducted at the Nottingham Holme Pierre Point National Whitewater Centre, on the top hole at the inlet gate. The participant completed a series of front loops. Video footage was obtained using a Casio EX-F high speed camera (operating at 300fps). The camera was located such that the calibration frame was set in the middle of the feature. The camera remained stationary during filming.
Initiation

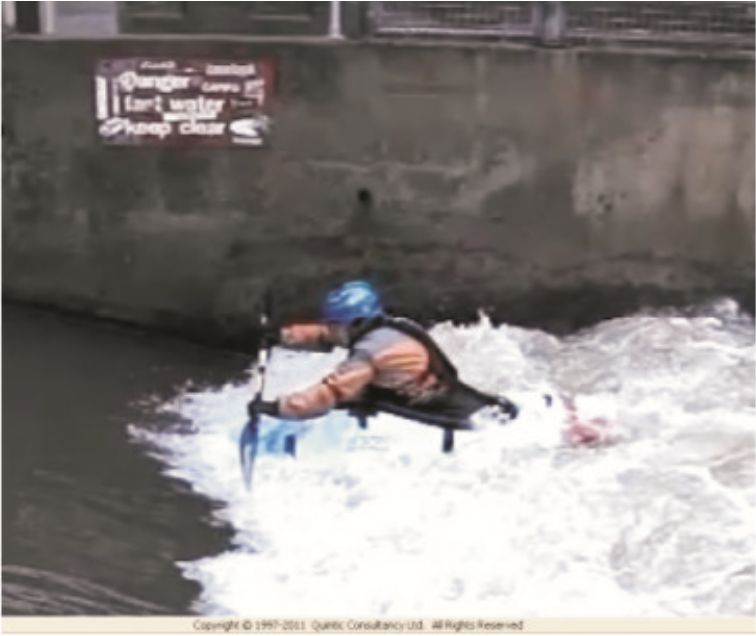

Figure 3 – Initiation position for loops 1, 2 and 3.
During initiation the athlete aimed to submerge the bow of the kayak to maximum depth. This was accomplished by throwing the mass forward and transferring this momentum to the kayak by pushing on the feet.
In loop 1 and 2 there was greater hip flexion as the outstretched arms (flexion) enabled the athlete to attain a smaller angle between knee and body 35.48º and 35.43º. This hip flexion was reduced in loop 3, due to the orientation of the arms and the angle between knee and body was increased to 48.28º. This increase in angle between body and knee reduced the resultant downward component of throwing the mass forwards and was one of the factors that reduced the maximum depth attained.
Maximum Depth
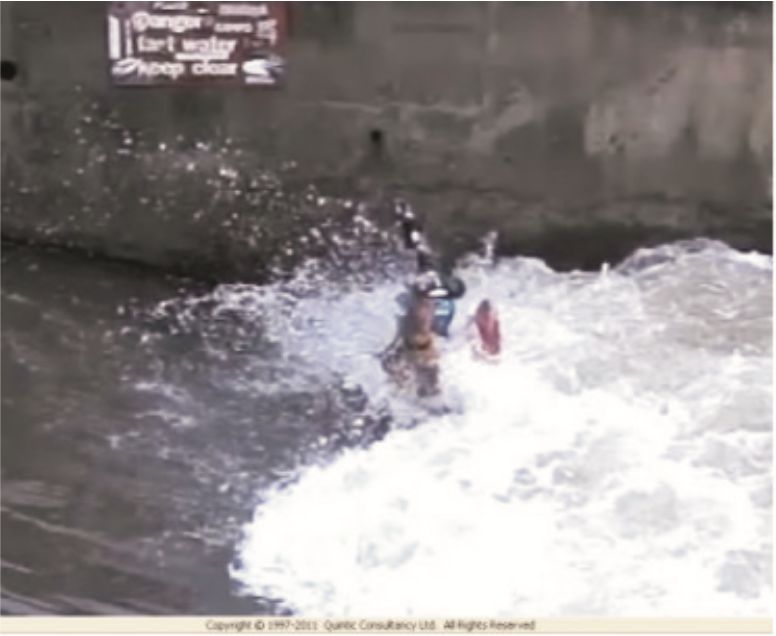

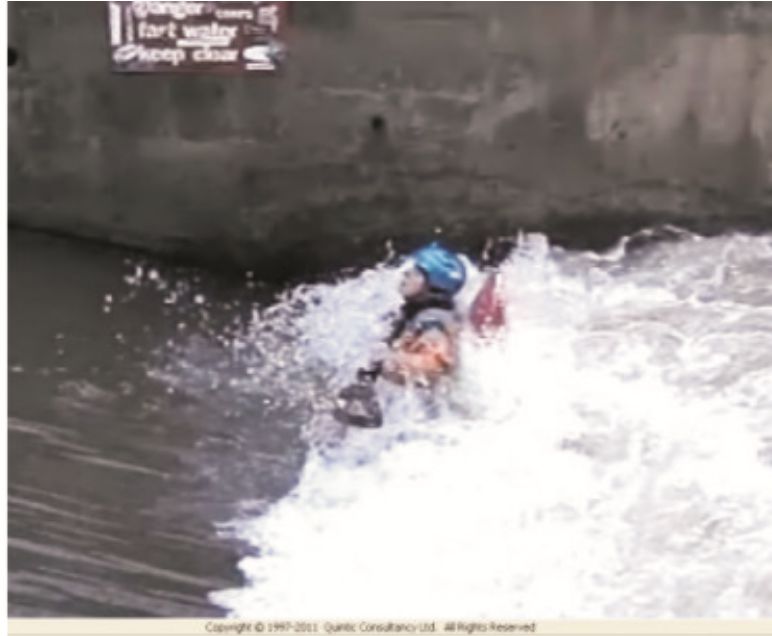
Figure 4 – Maximal depth for loops 1, 2 and 3.
Similar to diving, where the downward thrust by the athlete onto the spring board produces flexion in the sprung surface and the recoil of the flexed surface helps drive the athlete in to the air (Carr, 2004), the kayaker uses downwards thrust to submerge the kayak and the resultant buoyancy force drives the athlete into the air.
The maximum depth was measured for each of the three loops. Loop 1 and loop 2, both with the arms at full flexion at take-off, had similar maximal depths 1.38m and 1.37m respectively. This compared to a maximum depth of 0.87m for loop 3, where the arms were not used in take-off. This was expected as the arm swing during take-off provided momentum transfer to the athletes’ body (Carr, 2004). The action of the arms added to the downward thrust of the legs (resulting in a greater depth being observed) and increased the reaction force (buoyancy force) of water acting upward against the athlete/kayak.
The time from initiation to maximum depth (downwards motion) and from maximum depth to take-off (upwards motion) were calculated. For loop 1 and loop 2 the time to maximum depth exceeded the time from maximum depth to take-off. Miller and Munro (1985) reported similar observations for springboard diving, noting that the time for depression always exceeded the time for recoil.
Take-0ff



Figure 5 – Body position at take-off for loops 1, 2, and 3.
As the athlete approached take-off it was important that the upward thrust from the buoyancy force did not pass through the centre of gravity (CoG). The buoyancy force, acting out of alignment with the CoG, caused an eccentric force (f) about a distance (d) from the axis of rotation resulting in a torque (f x d).
The angle of the kayak at take-off was similar for loop 1 and loop 2, being 54.02° and 53.66° from the vertical and 58.96° for loop 3. This can be explained by the preceding motion. Here, the force of the water on the bow of the submerged kayak is rotating (positive rotation) the kayak downstream. In loops 1 and 2 the flexion of the arms increased the moment of inertia and slowed the rate of rotation, thereby giving a steeper angle at take-off.
The angular velocity at take off was calculated as 8.46rad/s (loop 1), 9.69rad/s (loop 2) and 9.31rad/s (loop 3). This was again related to body position. For loop 2 and loop 3 the position of arms (local to the body in the plane of rotation) resulted in the mass of the arms being more closely aligned to the axis of rotation (reducing the moment of inertia), thereby increasing the rate of rotation. The arm flexion in loop 1 had the effect of increasing the moment of inertia and reducing the angular velocity during flight. This is analogous to vaulting somersaults where it is noted that the sooner the athlete moves to the tucked position the sooner they will complete the required somersault (Takei, 2007).
Flight
The time of flight was calculated for the three loops as 0.17s (loop 1), 0.146s (loop 2) and 0.12s (loop 3), with the associated maximum elevations (highest point obtained by the kayak stern) achieved of 2.06m, 2.13m and 1.91m. The reduced elevation and flight time observed in loop 3 was related to the reduced maximum depth obtained in loop 3 and hence the reduced buoyancy force acting on the kayak to provide upward momentum. This when combined with the compact body shape in loop 3 ( figure 1, sequence 3) gave the observed reduction in flight duration.
Loops 1 and 2 have a similar maximum elevation as would be expected due to the similarity of maximum depth achieved and hence comparable buoyancy force providing upwards momentum.
Loop 2 had an increased rate of rotation, demonstrated by the angular velocity which was on average 14% higher than that of loop 1 for all positions considered. This increased rate of rotation observed in loop 2 can be explained by the compact body shape and the resultant lower moment of inertia than in loop 1. This compact body shape is clearly evident from analysis of the body motion in loop1 and loop 2; the angle from the arm to the body about the shoulder was 105.46º in loop 1 versus 16.52º in loop 2 and the angle at the elbow was 178.96º for loop 1 and 96.08º for loop 2.
Recovery

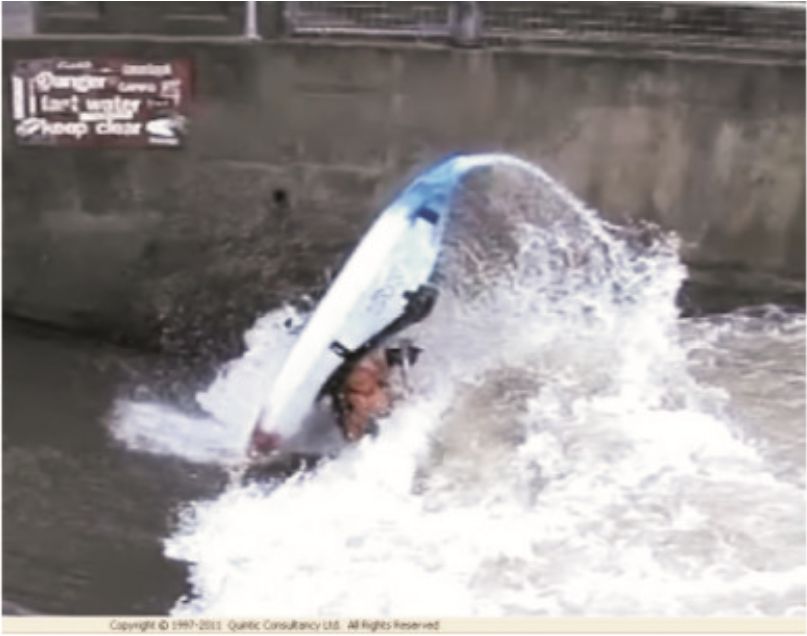
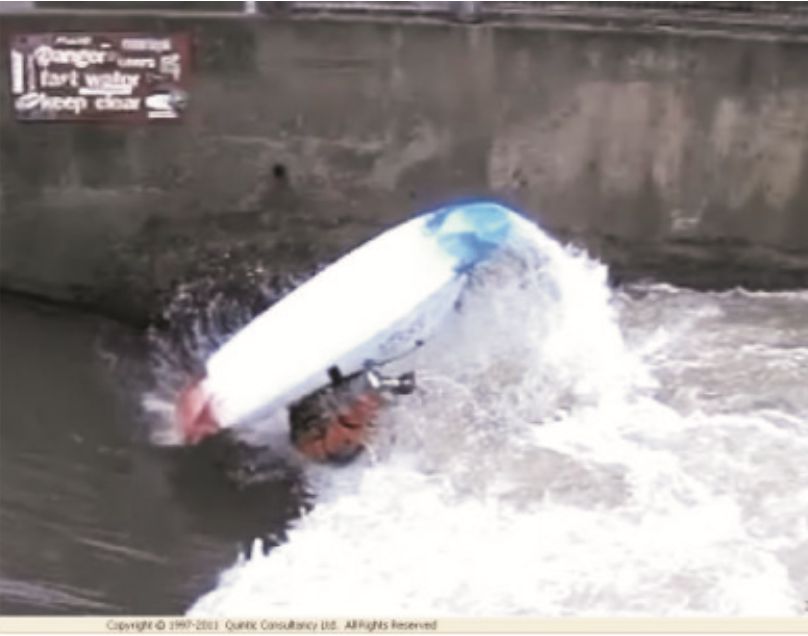
Figure 6 – Recovery for loops 1, 2 and 3.
Recovery described the point at which the kayak stern first touched the water on completion of the flight phase. The angle at landing was similar for loop 1 and loop 2 (217.69º and 217.06º respectively) and higher for loop 3 (231.11º) reflecting a shallower angle of entry. This shallower angle of entry was reflected in the shallower initial angle of take-off, already discussed, as the athletes flight path is set at take-off and cannot be changed in flight.
Discussion and Intervention
The study aimed to identify the key parameters and interventions required to execute the optimum kayaking front loop. Analysis of the techniques has identified that loop 2 is the optimum technique. This most closely mirrored that used by springboard divers (Sanders and Wilson, 1987; Miller and Munro, 1985).
For a successful initiation and to obtain maximum depth of kayak submersion the arms should be swung upwards (flexion) at the time the legs are thrust downwards during the initiation phase.
To initiate rotation the upwards thrust from the buoyancy force must not pass through the athletes centre of gravity. As the athlete approaches becoming airborne the body position is changed to bring the paddle in towards the chest, with the shoulder abducted to 90°, externally rotated to 90º (in the frontal plane) and the elbow flexed to 90º. This swinging of the arms and shifting of the head also assists in promoting rotation.
Adoption of this method in loop 2 resulted in the highest elevation of the three loops and a shorter flight time than loop 1 (which used the same initiation / take-off motion).
The athlete used for the study has a paddling style that more naturally mirrors that of loop 1 in terms of retaining arm flexion throughout the front loop. To improve the performance of the front loop it is proposed that the athlete adopt the approach used in loop 2. In order to assist the transition to the loop 2 motion it is proposed to conduct land based proprioceptive awareness activities as part of an integrated training programme (10 repetitions of the action prior to each training session). This will help to create links between the brain and the muscles being activated and make the mental pattern for loop 2 more natural. Combined with additional coaching and video analysis the transition to the new technical variant of the skill can be monitored.
Experimental Limitations
The experiment provided useful information into the biomechanical analysis of the kayak clean front loop. It identified several key characteristics of the movements required for execution of the optimum front loop. The experiment however had several limitations.
During filming it was not possible, at the venue chosen, to orientate the camera at the same elevation as the feature to be used. The video footage was therefore had a slight angle. The video footage was also difficult to interpret due to lighting conditions. It is suggested that artificial floodlighting should be used for future research.
The markers used on the kayak and athlete were sometimes difficult to interpret due to their being submerged, which restricted the availability of data. To obtain better data it is suggested that a skin tight dry-top should be worn to avoid movement of the markers and obscuring of markers by clothing.
References
Carr, G., (1997) Sports Mechanics for Coaches, Champaign, IL: Human Kinetics
Dempsey, W. T., Gaughran, G. R. L., (1967), Properties of body segments based on size and weight, American Journal of Anatomy, 120, 33-54.
Miller, D. I., Munro, C. F., (1985) Greg Louganis’ springboard takeoff: I: Temporal and joint position analysis, International Journal of Sports Biomechanics, 1, 209-220
Sanders R. H., Wilson, B. D., (1987) Angular momentum requirements of the twisting and non twisting 1.5 somersault dive, International Journal of Sport Biomechanics, 3, 47-62.
Takei, Y., (2007), The Roche vault performed by elite gymnasts, Somersaulting technique, Deterministic model and judges scores, Journal of Applied Biomechanics, 23, 1-11.
You may also be interested in:
3D kinematic analysis of Freestyle Kayaking: A comparison between manual and automated motion analysis techniques
You must be logged in to post a comment.